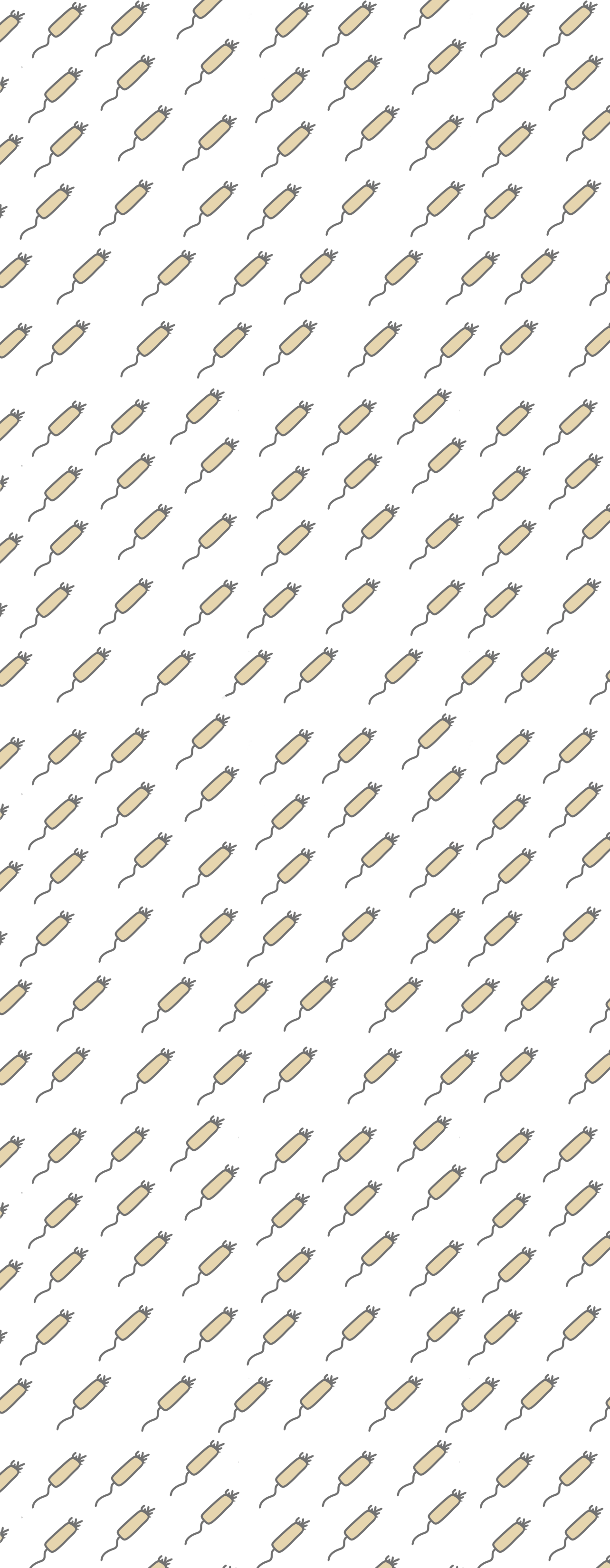
RESEARCH QUESTIONS
Pseudomonas aeruginosa is one of the leading causes of chronic infections due to its ability to form robust, antibiotic tolerant biofilms. It is therefore imperative to better understand bacterial biofilm physiology in order to discover new ways to dismantle biofilms and clear infections. Basic science discoveries regarding the physiology of bacteria will be the driving force to uncovering novel information on how bacteria mechanistically interact with their hosts. Our research focuses on understanding the regulatory role of acetyltransferases, which are hypothesized to influence biofilm development by modulating protein and small molecule activities. By analyzing the intricate regulatory mechanisms of acetylation, we seek to advance our understanding of how bacteria generate biofilms in their natural environments. Read more about acetylation and biofilms below:
What is acetylation?
Acetyltransferases are ubiquitous enzymes in all domains of life, constitute a diverse family boasting over 10,000 members and yet, despite the fact that they are increasingly found to play a pivotal role in the regulation of bacterial metabolism and physiology, less than 0.01% of bacterial acetyltransferases are characterized. Acetyltransferases use acetyl-CoA to modify the epsilon amino group of lysyl residues on proteins or primary amino groups of small molecules, altering their respective functions. Protein acetylation is reversible, with lysine deacetylases removing the acetyl-group and restoring the target's original function. This dynamic "on/off" switch is an ingenious adaptation by bacteria to tailor their physiology, ensuring survival in dynamically changing environments. In bacteria, a genome may encode anywhere from 20 to 80 putative acetyltransferases. The sheer number of these enzymes in annotated genomes indicates acetylation plays a critical role in the physiology of bacteria.
Schematic of protein acetylation and deacetylation (A) and small molecule acetylation (B). In A, the green protein is active, while the red protein is acetylated and inactive.
What are biofilms?
Biofilms are multicellular structures that are characterized by adherence of microorganisms to each other and their surfaces. Planktonic (single cells) generate essential components for initial attachment and microcolony formation of the biofilm. Overtime, an extracellular matrix encompasses the cells, which generates a biofilm that is resistant to mechanical perturbation. Mature biofilms have unique metabolic and structural features that confer resistance to external stressors (i.e. the immune system, antibiotics).
Like many bacteria, P. aeruginosa exists predominantly as a biofilm in the environment. Pseudomonas species are known to form biofilms within host tissues (e.g. wounds, the lungs of cystic fibrosis patients), on medical devices (e.g. implants, catheters), and in water sources (e.g. pipes). The genome of P. aeruginosa, encodes 44 putative acetyltransferases and a recent global transposon screen found that over half of P. aeruginosa acetyltransferases are beneficial to host colonization. We aim to unravel the role acetylation plays in the formation and maintenance of P. aeruginosa biofilms and will unveil novel regulatory events that contribute to our understanding of infections.
How are acetylation and biofilms connected?
If we can understand how biofilms form or disperse, we can understand the best way to dismantle them in vivo. While ~80% of bacteria in the environment exist in biofilms, the role of acetylation in biofilms is largely unexplored. We seek to explore regulatory mechanisms of acetylation that are required for biofilm formation in P. aeruginosa. As geneticists, we determine function by deleting or overexpressing acetyltransferase genes and searching for relevant biofilm phenotypes. With these phenotypes, we dig deep into the mechanism of the acetyltransferase. We identify the acetyltransferse target (the hardest part) and carry out physiological studies that inform why a target would be regulated by acetylation in the first place. For example, if a transcriptional regulator is acetylated, does acetylation abolish DNA binding and induce transcription of biofilm formation genes? If so, how and why does the regulator become acetylated, and can we use that information to better treat chronic infections? Pathogenesis is inferred by physiology, so while we take a basic science approach, our research has clinical implications. As there are 44+ acetyltransferases and four deacetylases encoded in the P. aeruginosa genome, we are not hurting for projects. Pick your favorite and go!
How can we take advantage of the information we learn in our research to develop novel drugs for chronic infections?
Once we understand the physiological regulatory requirements for survival in chronic wounds, we can begin to disentangle novel ways to disrupt pathogens in vivo. My postdoctoral project focused on this topic, and I was able to design an enzymatic therapeutic that targeted a core process required for biofilm maintenance. Through this disruption, Pseudomonas became more sensitive to clinical antibiotics. Current collaborations involve applying this enzyme therapeutic to chronic wound animal models to test its efficacy in vivo. As we discover new regulatory mechanisms of Pseudomonas biofilm formation (as outlined above), we will begin to develop novel therapeutics that target biofilm robustness. For example, does an acetylated small molecule contribute to biofilm formation? If so, can we discover or design a deacetylase that reverses the acetylation event and leaves Pseudomonas unable to build a biofilm or be vulnerable to antibiotics? As bacterial physiologists and basic scientists, we are aptly set up to use our knowledge to come up with creative ways to interrupt the homeostasis of the biofilm.